-
Development of Solar-Battery Microgrid The GTL received a grant from the CEC to develop a microgrid at the UC Davis RMI Winery using second-life EV batteries.
-
Nissan LEAF Batteries Donated by Nissan for this Project
Nissan, 4R Energy, and Sumitomo Corp. generously donated 20 Nissan LEAFs worth of second-life EV batteries. -
Battery Banks
NNissan LEAF batteries assembeled into battery banks in the lab. -
Testing a Second-Life EES Battery Bank
A modular battery bank being tested to ensure safe and reliable operation. -
Microgrid Architecture
The microgrid includes two buildings, two battery packs, and two solar PV arrays.
Announcement: If you’re a current/future UC Davis student and want to help with this project, Contact Us!
Tasks will include: controls communications development, energy management algorithm development, systems design and integration, failure modes analysis, utility grid operator communications for demand response and ancillary services… and that’s just what we thought of off the top of our heads.
The Demonstration Site
This project is supported by a grant from the California Energy Commission (CEC) under EPC-14-085‘Demonstration of community scale low cost highly efficient PV and energy management system’. We are building a microgrid system at the Robert Mondavi Institute’s (RMI) Brewery Winery and Food Science Building (BWF) to demonstrate advanced microgrid energy management systems combined with solar generation and energy storage. The RMI at UC Davis is a research community comprised of two departments and three research centers. There are 300 students and faculty working on developing grape vines resistant to drought and disease, analyzing the complex chemistries in wine that give each their unique sensory qualities and reducing the environmental impact of winemaking through green chemistries, reductions in energy and water usage, and microbial food safety.
Photos taken by the CEC during their visit on March 3, 2019. (Image is a link to their Flickr Album)
Images from the Microgrid
About the Grant
The project is designed to address the requirements of Assembly Bill 32, the California Global Warming Solutions Act and general need for assured access to reliable sources of energy. To do this, California must develop distributed renewable energy sources capable of providing clean, high quality and reliable energy for the community while also improving grid capacity and stability through peak load reduction and improved renewable to grid integration. The overall goal of this project is to demonstrate that the proposed microgrid system can reduce the BWF’s daily peak-time energy demand more than 10% (up to 87%).
Our Approach
To this end, we are building a smart Electrical Energy Storage (EES) system using retired Electric Vehicle (EV) batteries. The EES system will provide a cost effective and efficient energy storage solution for the BWF and its multiple community-scale renewable generators (solar PV arrays). The EES will act as an energy buffer to align the maximum solar energy generation with the RMI’s peak grid energy demand. In addition to the existing 120kW PV array installed on the BWF, a new 100kW array will be installed on the Jackson Sustainable Winery Building (JSWB).
Why build a microgrid in a winery? The wine industry, and food processing in general, also is excellent target for energy efficiency improvements. According to the U.S. Census Bureau, the U.S. had 1,956 wineries in 2007, where 971 of the wineries were in California, producing about 85% of all U.S. wine. According to a Lawrence Berkeley National Laboratory (LBNL) [1] study, the California winemaking industry consumes over 400 GWh of electricity annually, the second largest electricity-consuming food industry in California after fruit and vegetable processing. Much of the electricity used in winemaking goes to refrigeration for cooling and cold storage. The rest is used for compressed air, hot water, pumping, bottling line equipment and other processes. The energy use of wineries can be extended to the more general area of food processing. Food processing is the third largest energy user in California, the top agricultural state in the nation. According to CEC 400-2008-006-REV California’s Food Processing Industry Energy Efficiency Initiative: Adoption of Industrial Best Practices [2], California’s food processing industry generates over $50 billion in gross annual revenues, consuming more than 3,700 million kilowatt hours of electricity. The high energy intensity of food processing makes it an excellent target for energy efficiency measures, as even a 1% increase in efficiency could save 4,000 MWh of electricity, or $570,000 per year.
Why Us?
So, what is the Green Transportation Lab doing working on a solar-battery microgrid for a winery? The logic goes like this: Electric Vehicles have batteries, but we have to do something with those batteries once they are no longer suited for powering a vehicle (usually after losing 20-25% of their capacity). Thus we can use these batteries for a less demanding application, like energy storage from solar panels. As EVs gain popularity, the number of aged vehicle batteries will increase, posing recycling issues and making second-life applications more attractive. Second-life batteries will significantly reduce the system cost (to about 1/6) while providing only 25% lower performance. Using second-life EV batteries allows us to cost-effectively increase the capacity of the EES while reducing the environmental impact of EV batteries.
How Does Energy Storage Help?
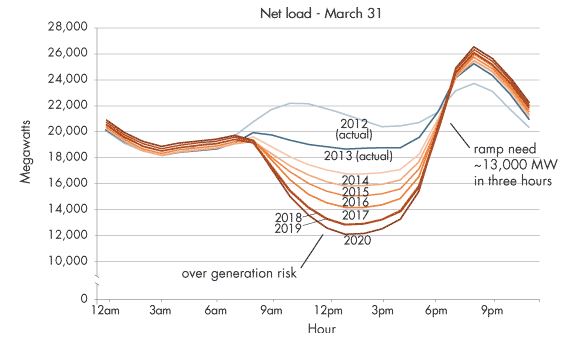
The CAISO "duck curve". Source [3]
In short, there are two (actually 3) problems: over generation during the day to a large amount of solar, and excessive ramp generation rate when the sun goes down. (Not to mention the intermittency of renewable resources.) Energy storage can help with all of these issues. During the day when there is potential for over generation, we store energy in our batteries. Then in the evening when solar power is fading, we can discharge the batteries to reduce both the peak demand. Thus fewer less efficient peaking power plants are required, the strain on the grid is reduced, and the ramp rate is reduced. And of course, energy storage can be used to level out the intermittency of renewable generation.
There is even a monetary benefit to the operators of large battery systems. By participating in the demand response (DR) program, they can be paid by CAISO to change their electrical usage on demand. This is difficult to do without an energy storage system that can be charged or discharged in accordance with the DR request.
The Second-Life Advantage
As discussed above, there are several advantages to installing energy storage in a microgrid. But what is the advantage to install used batteries over new ones? The major advantage is cost, lithium-ion batteries are still expensive, so utilizing “pre-owned” batteries significantly reduces the cost of energy storage systems. Additionally, as EVs and PHEVs become more popular (as they have been, especially in California [4]), the number of used batteries available will increase significantly. The cost benefit is not only to the second-life EES purchaser, but also to the automotive industry. If they can be sure to recoup a fraction of the battery’s cost even after it is no longer useful in their vehicles, the effective cost of EV batteries is reduced. This will either lead to more profits for them, or reduced prices of EVs (note there is quite a lot of competition in the EV market, so reduced price is likely).
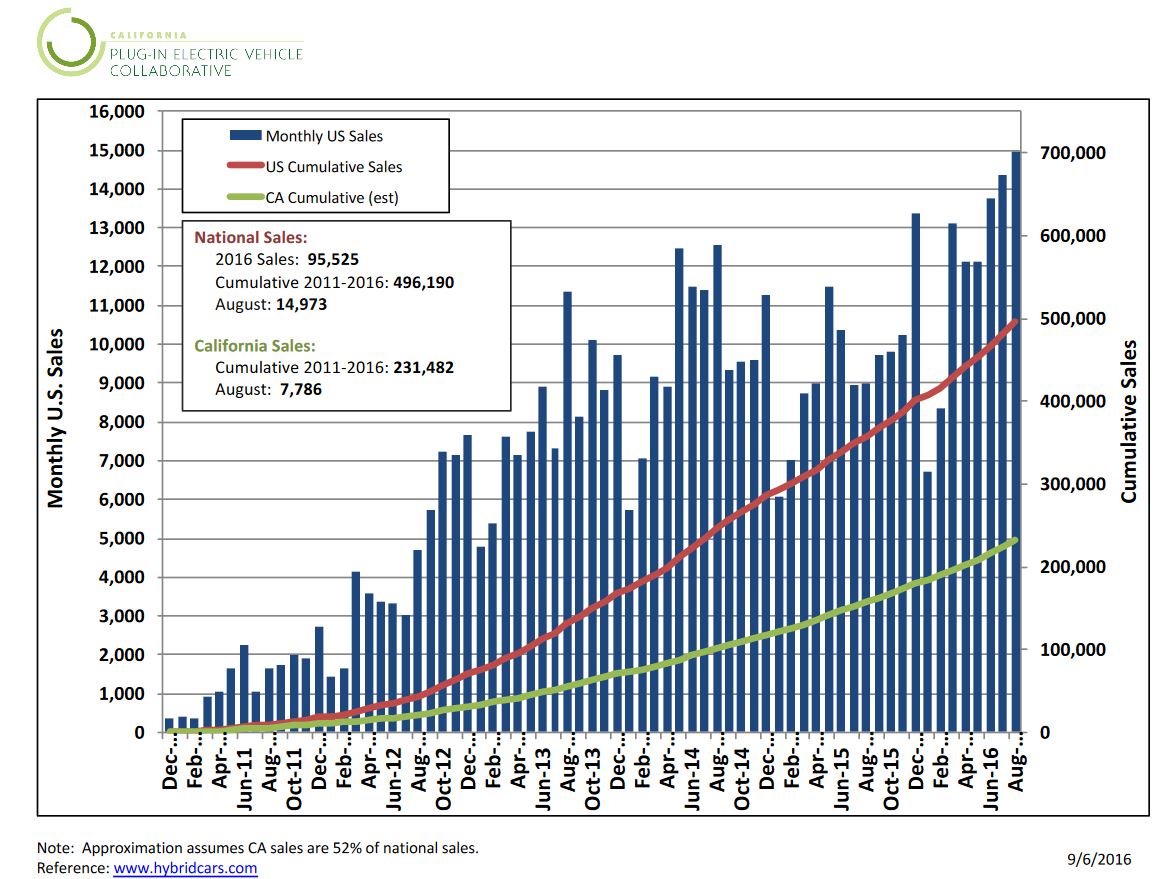
PHEV sales in the United States. Source [4]
The batteries in an electric vehicle are usually retired after their State of Health (SoH) reaches 75-80%. This means that when the batter can hold 75-80% of the energy that it did when it was new, it is replaced. Looking at it from another angle, this means that 75% of the battery’s capacity is “wasted”. By using these batteries for another purpose, the life-cycle environmental impact of EV batteries is reduced. Now, because the performance of the batteries is lower, they have a lower energy density than is ideal for a vehicle. This makes stationary applications, such as a microgrid, an ideal use for second-life batteries since energy density is not a large issue for stationary systems. (We don’t care how much energy it takes to get them going 60mph).
Another factor in battery degradation is that not all the cells in an EV’s battery pack will degrade in exactly the same way for a variety of reasons, including temperature gradients within the pack and tiny manufacturing differences. This means that the performance of second-life batteries can potentially be quite unpredictable. To address this issue, we designed our EES system to be modular, such that if we detect cells not performing well, we can easily replace them without disassembling the whole battery pack.
So, we’ve settled on using second-life EV batteries for our microgrid, how did we decide which manufacturer’s batteries to use? Our criteria were that the system had to be modular, and flexible enough to be disassembled and reassembled in a variety of form-factors and electrical configurations. We considered Chevy Volt, Tesla, BMW, Nissan, and Prius PEV batteries. Of these, we determined that the Nissan (LEAF) batteries provided the ease of use required, in terms of both electrical and physical size. The modules are small enough to be stacked in a variety of ways, and their voltages are small enough to be highly configurable to any desirable system voltage and capacity. Additionally, as one of the bestselling EVs on the market, there are many second-life batteries available, and there will only be more in the future. We contacted Nissan USA to discuss using their batteries for this project. They generously donated around 20 LEAFs worth of second-life battery modules to our lab for integration into the microgrid.
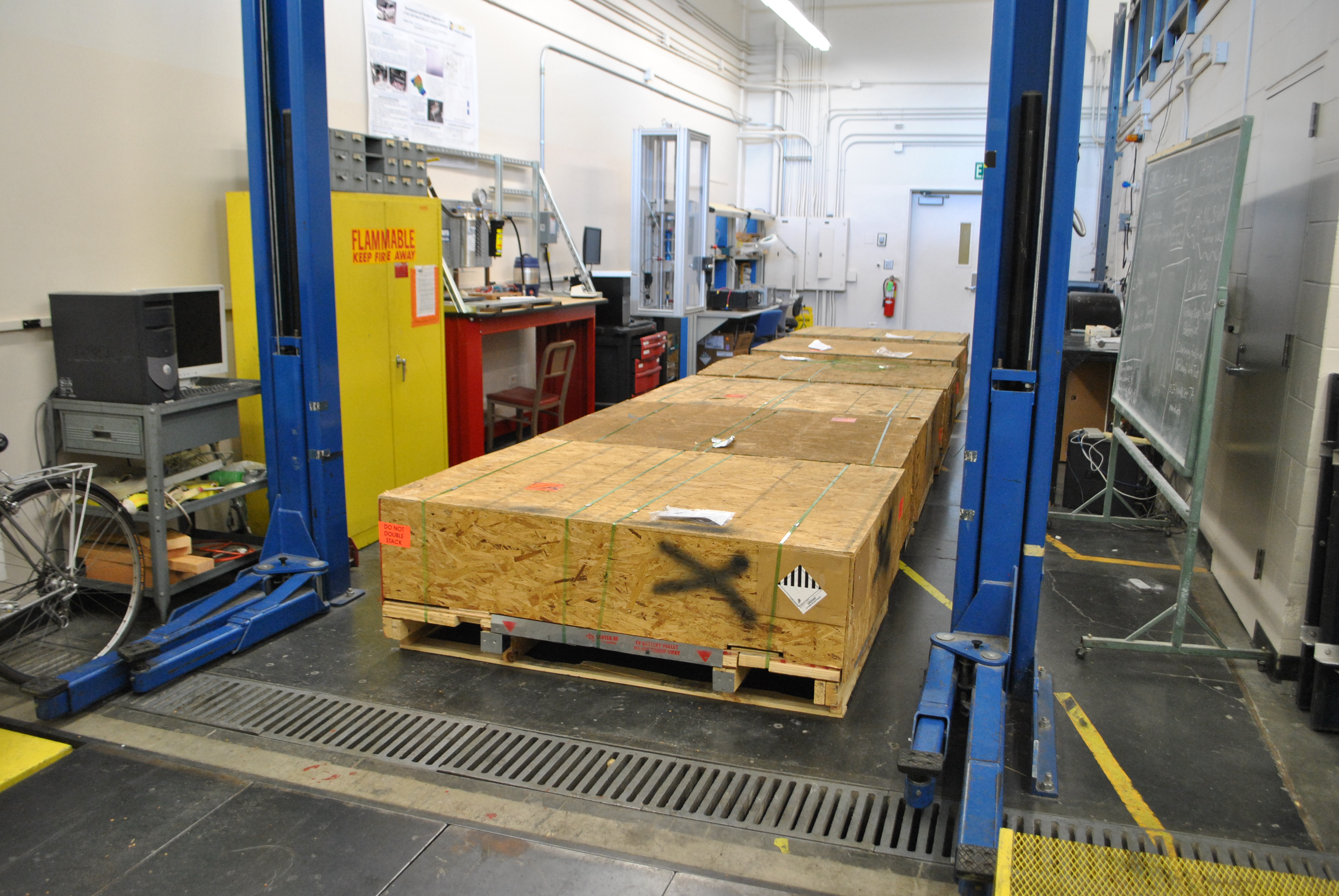
Shipment of Nissan LEAF batteries, courtesy of Nissan, 4REnergy and Sumitomo Corp.
The Microgrid System
The system consists of the BWF and the JSWB, a sub-circuit of the RMI. Both the BWF and the JSWB will have large PV arrays. The BWF currently has a 120kW PV array installed, while the JSWB will be getting 100kW of PV in the near future. The EES will be installed in the JSWB, and be DC/DC coupled through a multi-input inverter. This will allow for efficient charging of the EES from PV during the day from solar power so that it can be used when demand is highest. The EES is not large enough to provide for the entire load of the BWF without the aid of PV, so the system is not islandable and will still draw power from the utility grid at night. The BWF is connected to the RMI grid through its own meter, so any “over-generation’ from PV in our microgrid will be used by other buildings at UC Davis, but will appear to the microgrid as if power is being fed back to the utility.
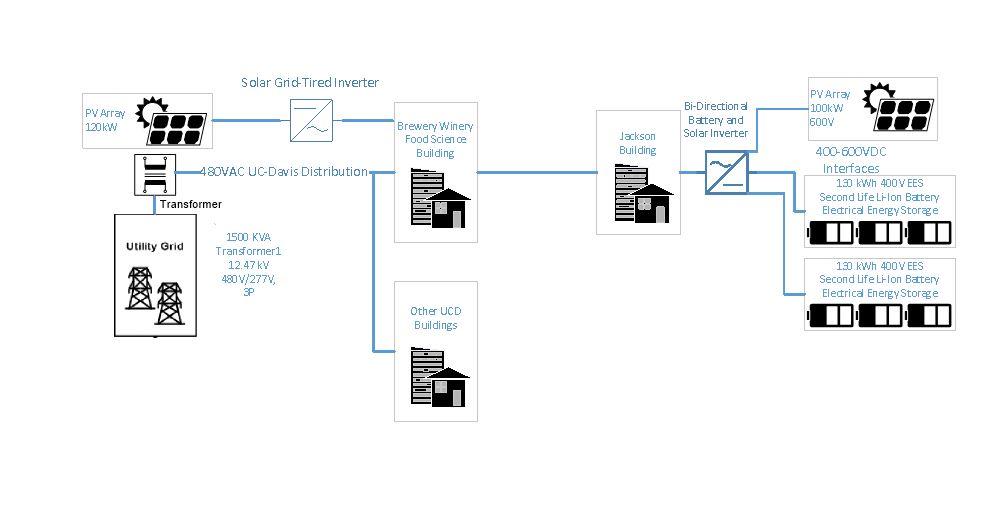
Diagram of the system to be installed.
The plot below shows the current electrical demand of the BWF compared to a simulation of the demand after the system has been installed. We can see that due to the extra PV, the building over-produces ~60kW during the summer, and that we can store about half of the daily solar over-generation in the EES. The power remains flat at zero while the EES is charging, but once full that power now goes to the grid, resulting the over-generation spike. The energy management system will be written such as to avoid this situation. In the evening, the EES discharges to keep the building’s load on the grid at a constant, low value during peak-hours, finally fulling relying on grid power later in the night when power is cheap. We can also see that, on the last day plotted, the availability of the EES was successful at smoothing out the variable load drawn from the grid, improving grid stability.
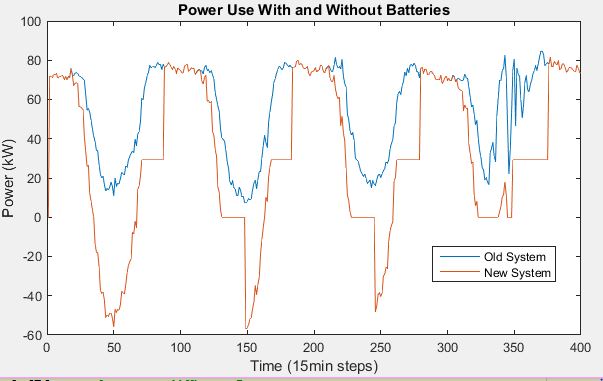
Estimated demand profile after microgrid is installed compared to before installation.
The plots below show the monthly electrical cost and CO2 associated with grid power (~0.28 kgCO2/kWh) for the current system, a system where we only install PV, and a system with PV and an EES. We can see that the scenario with the EES performs the best, but notably does not make as significant a reduction as the PV, especially to the cost. This is due to the highly discounted rate at which UC Davis purchases power. For residential customers, the cost savings more than double. For customers on a ToU (Time of Use) rate, the savings would be significantly more, as we can see from the figure above showing the significantly reduced demand during peak hours.
Another thing to note is that, for both cost and CO2 reductions, the EES helps the most during the summer. This is because the EES is not a power generator, and thus can only be as clean or cheap as the power used to charge it. During the summer, there is significantly PV over-generation which is used to charge the batteries, making their carbon footprint zero, and their electrical cost a function of the lifetime of the PV panels. However, during the winter, there is less PV, so some grid power is used to charge the EES, increasing both the cost and carbon footprint.
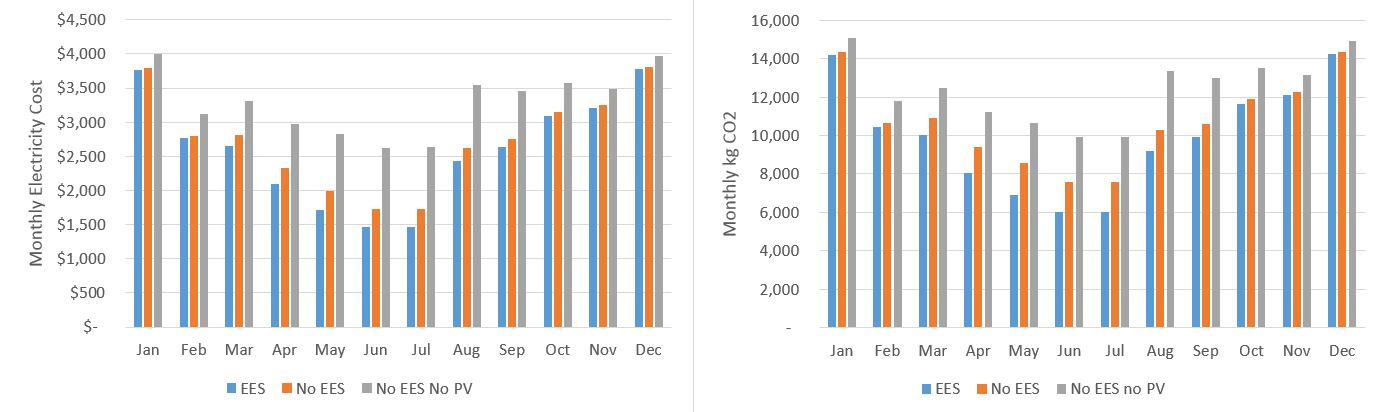
Estimated cost and CO2 savings resulting from installtion of the microgrid.
However, the savings can be increased further. For cost, by participating in DR, the BWF would be paid to reduce (or increase) its electrical load on CAISO’s command. This adds significant value to the EES. In terms of CO2 reductions, the renewable mix on the utility grid is not constant. Using data from CAISO, the EES could be charged at times when the mix of renewables is maximized, then discharge when the grid has a low renewable mix. This increases the renewable power utilization of the microgrid, and reduces its electrical carbon footprint.
[3] CAISO. (2014). Renewables Integration. Online at http://publications.caiso.com/StateOfTheGrid2014/RenewablesIntegration.htm
[4] Plug-In Electric Vehicle Collaborative, 2016, “Sales Dashboard”. http://www.pevcollaborative.org/sites/all/themes/pev/files/8_aug_2016_Dashboard_PEV_Sales.pdf
[5] View the BMS profile used for the battery system here: https://research.engineering.ucdavis.edu/greentech/rmi-microgrid-bms-profile/