Introduction
In the battery lab, we study the behavior lithium-ion batteries of varying chemistries under different conditions. Using this data, we create models, new test procedures, controls, and design systems that take advantage of high energy density storage. Thus, our lab combines mechanical design and analysis, electrical design, thermodynamics, heat transfer, energy systems, and machine shop skills to build “real-world” systems. What we do not do is design or fabricate battery cells. For a detailed view of what we do in the lab, check out the Publications tab and see our research and projects below.
Current Research and Projects
- Solar-Battery Microgrid at UC Davis
- Sustainable City of Dubai Grid Modeling
- Temperature Gradients in Parallel Cells
Past Research and Projects
- Lithium Polymer Studies
- Energy Storage for the Smart Aggie House
- Life Cycle Analysis of Cycling in Davis, CA
- Calculating Communicating Emissions at the UC Davis Medical Center in Sacramento
- Measuring the Effectiveness of the Green Commuter Program at UC Davis Medical Center in Sacramento
- Demonstration Nanogrid at UC Davis Picnic Day
Opportunities for Students
The battery lab welcomes new students to join us, we have plenty to do! While working in the lab, students will be exposed to a variety of tasks/activities relating to energy storage systems, microgrids, electric vehicles, and energy management. We projects include controls communications development, energy management algorithm development, systems design and integration, failure modes analysis, utility grid operator communications for demand response and ancillary services… and that’s just what we thought of off the top of our heads. Working in the GTL battery lab is a great way to learn to use multiple skills in a single context. If any of this sounds interesting to you, send us an email!
An Introduction to Lithium-ion Batteries
Why study Lithium-ion batteries?
Lithium ion batteries are a critical component of modern life, relied on by billions of people to power devices from the smallest health implants to large trucks, and even planes. They can be found in our phones, power tools, laptops, cameras, cars, aircraft, tablets, and the electric grid, to name a few. Despite their prevalence, lithium-ion batteries are a relatively new technology that was first commercialized in 1991. [1] This shows in the repeated incidents of commercial products becoming fire-hazards due to their lithium based batteries. In order to make these products safer, our understanding of these batteries must improve, and there are uses of these batteries that are only recently becoming feasible and study-able on a large scale. No device that stores large amounts of energy can be completely safe, this includes your car’s gas tank, flywheels, dams, or hydrogen fuel cells. However, the risks, with sufficient understanding, engineering, and protocols can be significantly reduced as shown by that last link. Thus the study of lithium ion batteries and their control systems is critical to ensuring consumer and public safety, especially when these devices are placed on/in aircraft and other vehicles.
How Batteries Work?
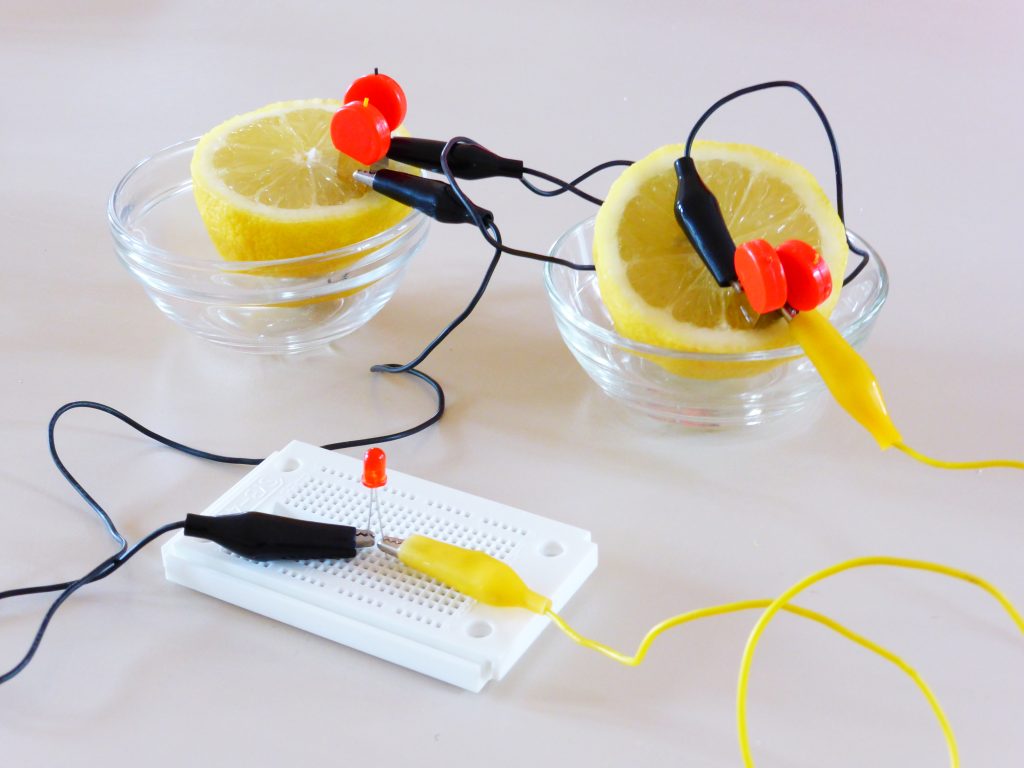
The inside of a battery is split into two sides, the anode and the cathode, by a separator that allows the passage of ions, but not electrons. On each side of the separator, a Redox half reaction takes place. For one of the reactions to occur, the other reaction much occur, since they ‘feed’ each other. These reactions generate the voltage that the battery provides, and the rate at which the reactions can proceed dictates the maximum amount of current that can be extracted from the battery. By only allowing ions to cross the separator (the name-sake lithium-ions in lithium batteries), the electrons that were stripped from the ions are forced to travel through an external circuit (your phone) to reach the other side of the cell, completing the other half reaction. This reaction looks something like this: [2]
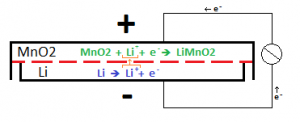

Characterization of Batteries
While many batteries will specify “The” voltage at which they operate, batteries do not work at constant voltage. The figure below shows a typical operating voltage curve for a lithium ion battery. It shows that the voltage decreases as the battery is discharged, and that the useable capacity is reduced if we discharge it faster so that the ‘real’ capacity is not always equal to the nominal advertised capacity, even for a new battery. Also, the starting voltage of these batteries was the same, but the one that was discharged faster had a lower operating voltage.
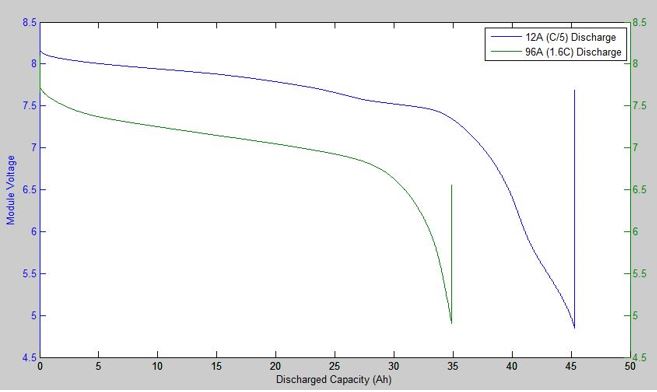
If you look closely, right at the start of the discharge, the blue and the green curves are closer together in voltage than further from the start of the discharge. The first ‘instantaneous’ voltage drop is due to Ohmic resistance, where the voltage drop is calculated by V=IR. We can use this relation to calculate the internal resistance of the battery due to resistive elements in the battery such as the current collectors and the terminals. The figure below shows the voltage (red) and the current (blue) of a battery. There was a 10 second pulse discharge, and we can see the response of the battery’s voltage. Not that it initially drops ‘instantly’ with the current pulse, then slowly continues to decrease. This second slower decrease in voltage is due to factors relating to the chemical reaction in the battery, notably the concentration of reactants near the current collectors.
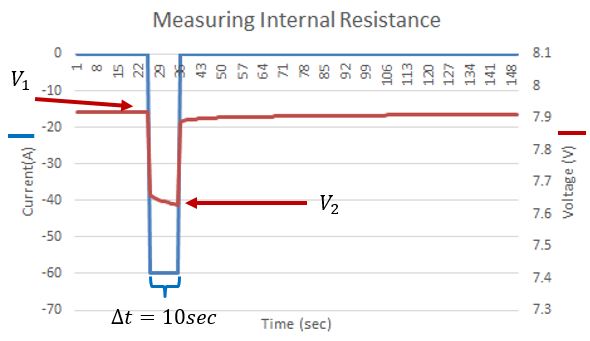
Current and state of charge are not the only factors affecting the voltage of battery. The plot below shows the dependence of the internal resistance of a battery on temperature, which as shown above has a direct effect on the operating voltage of a battery while under load.
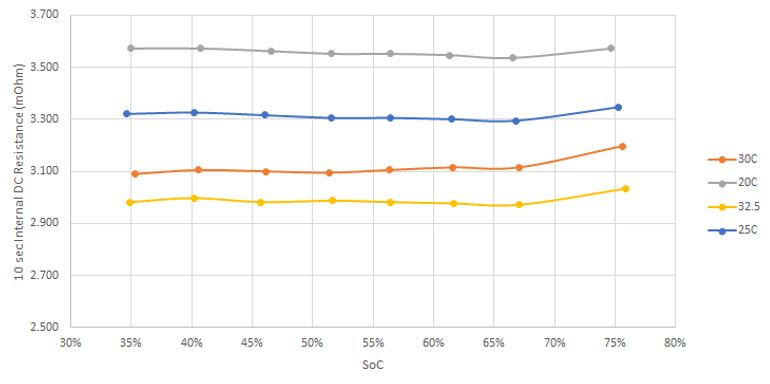
Lithium-ion Battery Safety
There’s the obvious one, don’t put a screw driver between the terminals. Doing so will result in two of the other most dangerous things to do with a lithium ion battery. The high current, thanks to P = I^2R will result in significant heating of the battery. Applying a large amount of heat (aka high temperature) is extremely dangrous. When the internal temperature of the battery reaches ~70 degrees C, the solid-electrolyte interface will begin to break down. This exposes the electrolyte to the metals in the anode and cathode, resulting in an exothermic reaction, adding to the heat in the battery. Left alone, this will result in thermal run-away, at which point it is not possible to cool the cell fast enough to counteract the heating caused by the reactions within the cell. The lithium in the battery will begin reacting with the electrolyte, producing flamible gases, this causes cells to expand or ‘ballon’ as shown in the picture below. Eventually, this can result in the cell rupturing, spewing its contents into the environment, and causing fires.
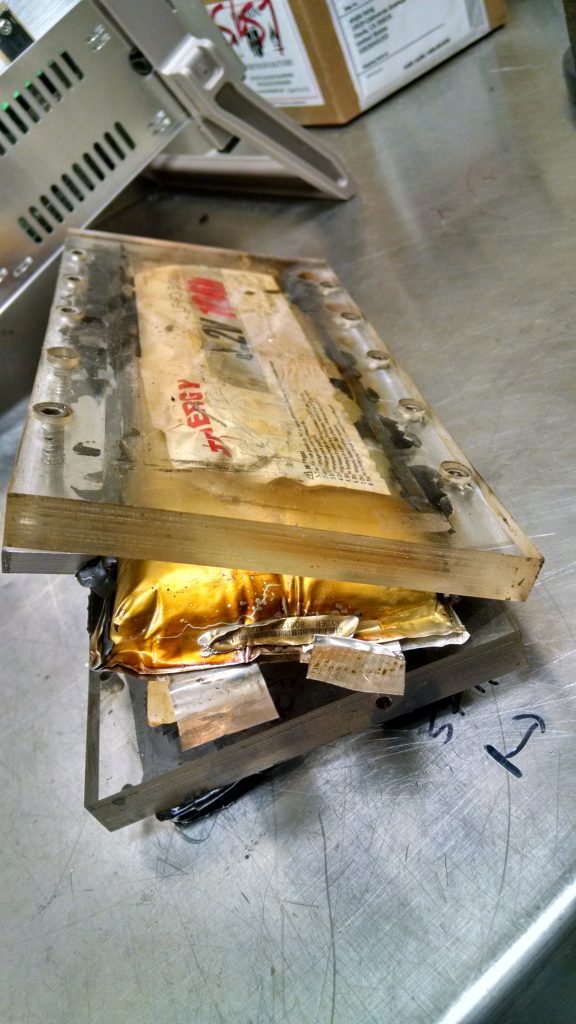
Left short-circuited for long enough, it will also result in over-discharge of the battery, which, while not as dangerous as over-charging, is not exactly desireable. Overcharging has a similar effect to excessive heating, since the cell will get very hot when over-charged, with the higher voltages within the cell promoting reactions to occur faster. Since there is more stored energy in the cell when it is fully charged, this can be very dangerous.
These safety considerations are why lithium batteries require battery management systems (BMS). A BMS’s job is to ensure that the battery is not subjected to excessive temperatures, over charging, over discharging, high current, and to protect against cell failures. It does this by measuring the voltage of every cell in the battery pack individually, and communicating with the chargers/loads to ensure they do not push or draw current when it is not safe. The BMS also has temperature control capabilities, it can be connected to a temperature regulation system to prevent the battery from leaving its safe operating region. A BMS also calculates ‘things’ about the battery such as its state of charge (SoC) aka the percentage bar, the state of health (SoH) of cells and of the pack (measured capacity / nominal capacity), internal resistance of the battery cells, and maximum safe currents for charge and discharge based on the battery’s current state.
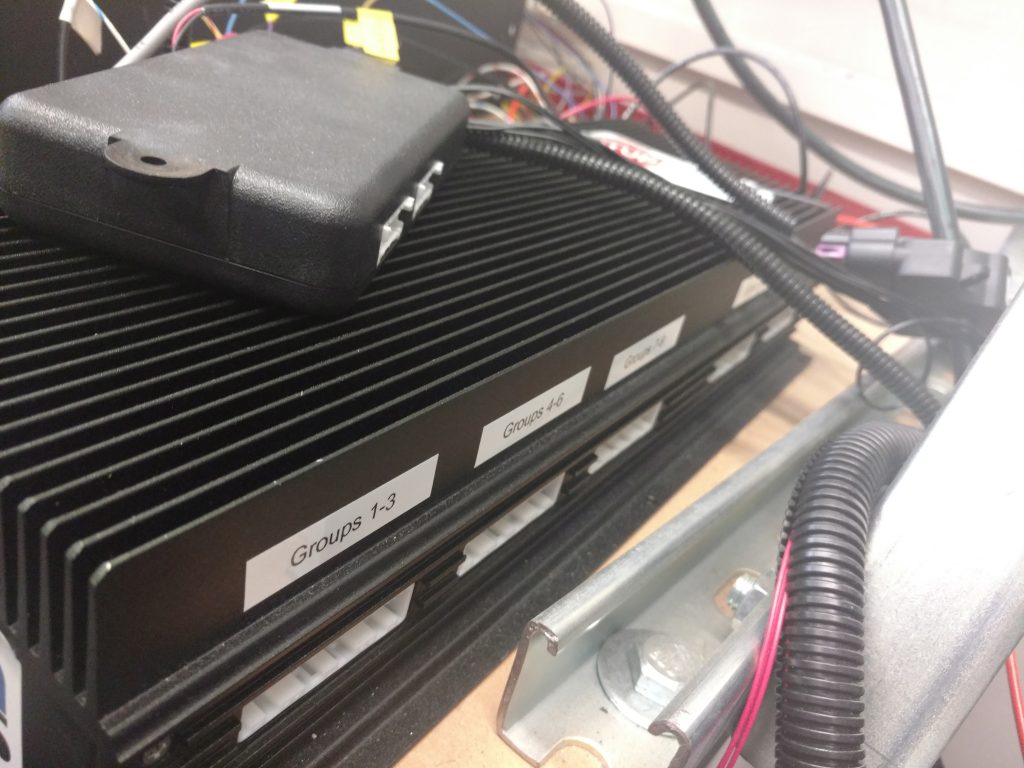
Facilities
The GTL Battery Lab is located in 1235 Bainer Hall.
The BATtery Cave: We have three Arbin Instruments battery test stations. These test stations are used for cell characterization, electric vehicle drive-cycle simulations, and long term degradation studies. The test station in the picture is a 12 channel system being used to test electric vehicle batteries.
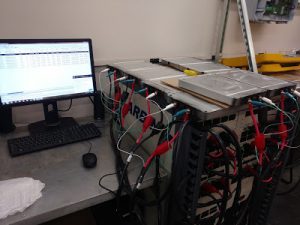
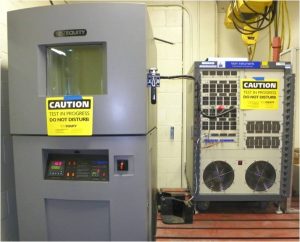
Left: The temperature chamber can be used to simulate various dynamic or static environmental conditions. The performance and degradation of lithium-ion batteries is strongly dependent on temperature, making known temperatures an important part of any experiment. Right: Another battery test station. This one has only one channel.
The grid-tied inverters we use to test battery packs for home-energy storage or industrial scale applications.
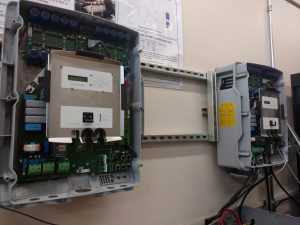
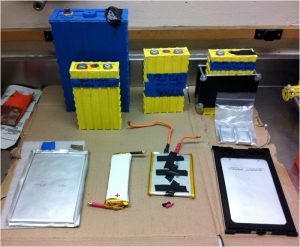
We test various form factors and chemistries of lithium ion batteries such as laptop batteries, tablet batteries, and electric vehicle batteries. Pictured: prismatic and pouch cells. Prismatic cells are encased in hard plastic to improve their durability, while pouch cells are protected only by a thin film which increases their energy density relative to prismatic cells.
[We no longer have this EV.] The lab’s “(Lack of) Electric(ity) Vehicle”, AKA ZENN (Zero Emissions No Noise) NEV (Neighborhood Electric Vehicle). Currently not functioning due to its lack of batteries. An upcoming project will be to replace the lead-acid batteries it was designed to operate on with Lithium-ion batteries and the appropriate circuitry to protect them from exploding, as a certain phone has been known to do.
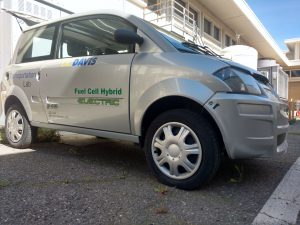
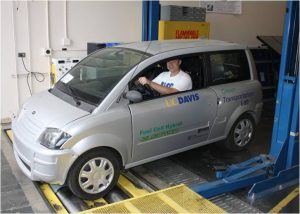
Test dyno for (electric) vehicle studies. This picture is not presently possible, since the ZENN only moves if we push it. The dyno however still works, probably. We haven’t used it for years.
[We also no longer have this.] This is our engine dyno mounted to a rotary engine for series hybrid powertrain studies. The engine dyno was used by the formula hybrid team when they occupied this lab. The computers that control it run on Windows XP, so are not permitted to be connected to the internet.
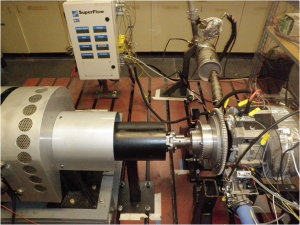
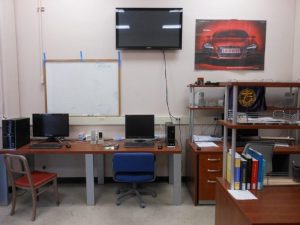
A picture of the student office attached to the lab. It has high speed computers for computational modeling and data analysis (but not the ones in the picture, a universe does not exist where those are fast computers). The lab also has a mini-fridge and coffee maker without a supply of coffee.
Thermal imaging to collect data on battery performance, check experimental set ups, ensure wiring is well connected, and because it’s cool. The image shows three busbars connected to a battery pack.
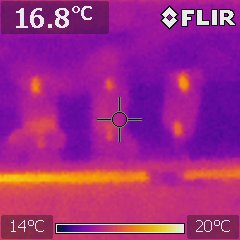
References
[1] Sony Keywords to understanding Sony Energy Devices. http://www.sonyenergy-devices.co.jp/en/keyword/
[2] Wang, Q., Ping, P., Zhao, X., Chu, G., Sun, J., & Chen, C. (2012). Thermal runaway caused fire and explosion of lithium ion battery. Journal of power sources, 208, 210-224.