View the report here.
The Demonstration Site
The Sustainable City in Dubai (SCD) is expected to be the first residential community in Dubai meeting the highest environmental standards by adopting a sustainable approach including photovoltaic (PV) solar production and electric vehicles (EVs).
About the Project
While PV solar production helps the city to generate energy with little detriment to the environment, the intermittent nature of solar generation requires special attention when connecting to the grid. Critical issues include grid instability and the imbalance between energy demand and production, especially during periods of over-generation. The SCD is equipped with a significant volume of PV panels, capable of producing up to 10 MW. However, the grid lacks electrical energy storage (EES) and an energy management system (EMS). Integration of EES and EMS will provide the SCD with additional benefits including: 1) significant reduction of peak demand, 2) improved power quality and stability, 3) island-mode operation in case of blackout, and 4) enhanced sustainability of the EVs by charging them preferably with the solar electricity stored in the EES.
As the penetration of renewables and demand for a secure and stable grid continue to grow, so, too, does the need for grid-connected electricity energy storage in order to maximize the sustainability, reliability and independence of renewable microgrids [1, 2]. Although pumped hydro is a cost effective (less than $100/kWh), durable and efficient (>70% round trip efficiency) EES solution, which accounts for 99% of grid storage systems operated in the U.S. [3], its deployment requires specific geographic location, and imposes significant environmental impact. By contrast, electrochemical storage systems show the most promise as they have the advantages of localization flexibility, scalability, and efficiency. Popular candidates for electrochemical storage include lithium batteries, lead acid batteries, flow batteries and sodiumsulfur high temperature batteries [4]. Lead acid batteries a nd flow batteries have lower energy density and efficiency, but are more cost-effective [5]. Sodium-sulfur batteries have high energy density, but have high production cost and require infrastructure for safety assurance [3]. Lithium batteries have high power and energy density, but have high production cost and require battery management circuitry. Second-life lithium batteries (i.e., those that have been used for several years) are gaining appeal for EES applications since they provide compatible performance at a reduced cost [6], and repurpose an otherwise wasted resource. In all, as opposed to centralized bulk storage such as pumped hydro and compressed air, small-scale battery systems provide a decentralized, scalable, and more responsive EES solution, which addresses the needs for a microgrid to perform demand response and dynamic peak shifting. Furthermore, proper modeling and simulation of an EES solution can identify ways to avoid common pitfalls and maximize sustainability and reliability [7].
Our Approach
The overall goals of this project are to: 1) develop a computer model of the SCD microgrid with EES and EMS, taking into account user behavior and preferences; 2) simulate the grid operation with proposed EES and EMS to enhance grid stability as well as to minimize the peak demand; 3) design and simulate the SCD grid for higher-level of sustainable scenarios, including off-grid mode; and 4) carry out the performance evaluation of the proposed system.
The first tasks for this project will be data collection for the grid architecture of the SCD and the characteristics of loads and user demands. We will gather metered energy consumption data, and conduct an energy use survey to have a better understanding of energy consumption of residential buildings and commercial buildings, and EV charging patterns.
In the next step, we will develop a numerical model of a subdivision of SCD incorporating PV panels, EES and EV charging stations. The developed model will be validated by grid operation data from the UC Davis Aggie House and the Robert Mondavi Institute (RMI) microgrid. The model will simulate SCD grid operation based on local solar energy production and energy demand data including EV operation as well as residential and commercial buildings. The grid performance will be analyzed in terms of energy cost, peak shaving and environmental impacts. The results will identify the optimal design of the EES and EMS for the SCD microgrid. In the next step, we will redesign and optimize the SCD microgrid with additional renewable generation capacity and EESs for a higher level of sustainable operations, such as demand response (DR), trading overproduction and off-grid operation. Finally, a comprehensive assessment will be performed including system performance and economic analysis such as payback period of the proposed system. Together, the technical, social, and economic outputs of this research will substantially improve sustainability, stability, and reliability of the electrical grid of the SCD.
How Does Energy Storage Help?
Let’s start with California’s electricity demand profile. While not Dubai’s demand, this is a typical load profile. The figure below is from the California Independent System Operator (CAISO), they’re the ones who operate the overall electrical grid and power flowing through it in California. Since California has installed so many solar panels to meet the state’s RPS requirements, during the day conventional power sources produce less power, which is good since it reduces CO2 emissions. On the other hand, if there is too much renewable power, generators such as nuclear plants that cannot quickly change their output risk over generation, which is dangerous for grid stability. Later in the day when the demand for electricity is greatest, solar panels stop working. This means that conventional generation which was generating less than it otherwise would have been needs to both replace the rate at which solar power fades and the rate at which demand is increasing. This makes conventional generators need to ramp up extremely quickly, and some may not be able to do so. To account for this, generators that are faster to respond, but also less efficient are used, resulting in extra pollution. [9]
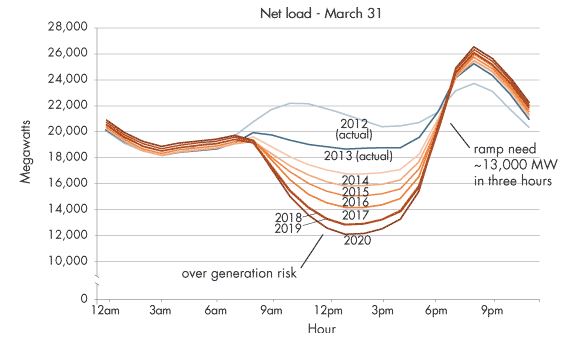
The CAISO "duck curve". Source [3]
In short, there are two (actually 3) problems: over generation during the day to a large amount of solar, and excessive ramp generation rate when the sun goes down. (Not to mention the intermittency of renewable resources.) Energy storage can help with all of these issues. During the day when there is potential for over generation, we store energy in our batteries. Then in the evening when solar power is fading, we can discharge the batteries to reduce both the peak demand. Thus fewer less efficient peaking power plants are required, the strain on the grid is reduced, and the ramp rate is reduced. And of course, energy storage can be used to level out the intermittency of renewable generation.
There is even a monetary benefit to the operators of large battery systems. By participating in the demand response (DR) program, they can be paid by CAISO to change their electrical usage on demand. This is difficult to do without an energy storage system that can be charged or discharged in accordance with the DR request.
[1] N. Motegi, M. A. Piette, D. S. Watson, S. Kiliccote, and P. Xu, Lawrence Berkeley National Laboratory LBNL-59975, 2007.
[2] C. A. Hill, M. C. Such, C. Dongmei, J. Gonzalez, and W. M. Grady, Smart Grid, IEEE Transactions on, vol. 3, pp. 850-857, 2012.
[3] A. Poullikkas, Renewable and Sustainable Energy Reviews, vol. 27, pp. 778-788, 2013.
[4] B. Dunn, H. Kamath, and J.-M. Tarascon, Science, vol. 334, pp. 928-935, 2011.
[5] S. J. Tong, A. Same, M. A. Kootstra, and J. W. Park, Applied Energy, vol. 104, pp. 740-750, 2013.
[6] Mark A. Kootstra, Shijie Tong, Jae Wan Park,’ Photovoltaic grid stabilization system using second life lithium battery,’ Int. J. Energy Res. 39 (2015) 825–841
[7] Xia, Linyi; Dichter, Nelson; Woolley, Jonathan, Modera, Mark; Smedley, Keyue; Li, G.P. (University of California, Irvine, California Plugload Research Center, University of California, Davis, Western Cooling Efficiency Center). 2015. Smart Power for Smart Home: Power Factor Correction, and Peak Demand Reductions. California Energy Commission. http://wcec.ucdavis.edu/wp-content/uploads/2015/09/Smart-Power-Smart-Home-FinalReport.pdf
[8] D. H. Doughty, P. C. Butler, A. A. Akhil, N. H. Clark, and J. D. Boyes, The Electrochemical Society Interface, pp. 49-53, 2010.
[9] CAISO. (2014). Renewables Integration. Online at http://publications.caiso.com/StateOfTheGrid2014/RenewablesIntegration.htm